Towards a better understanding of lymph circulation
Catherine PONS-HIMBERT*,
and Elisabeth LAEMMEL**
**Université Paris 7
Paris, France
SUMMARY
The lymphatic system was for years considered an “accessory” system and was neglected in comparison with the vascular system, which appeared much more crucial. In the last decade, researchers have become interested in lymphatic function since many diseases seem to interact with it (cancer, inflammation, infection, auto-immunity). The lymphatic system is harder to study than its vascular counterpart as its vessels are ill-defined, almost invisible. Intravital microscopy alone correctly visualizes these structures, thus shedding light on their function and quantifying their movements. This paper focuses on lymph anatomy and physiology, summarizes research trends, and considers lymph diseases and the latest treatments, particularly of cancer.
INTRODUCTION
The circulations of both blood and lymph are involved in cardiovascular function. Blood circulation is a closed circuit, but many exchanges occur at the venular and capillary levels between blood tissue and perfused organs. Fluid and proteins can cross from one compartment to another. The lymph circulation returns the lost fluid to the general circulation.
Two etiologies can be distinguished in lymph disease: excess fluid in the interstitium due to changes in permeability, and impaired draining of fluid by the lymphatics.
Lymphedema results from fluid accumulation in the interstitial compartment of the extravascular space.1 Although frequently encountered in medical practice, its prevalence is not well established, because we lack a precise definition, treatment varies, and affected populations are poorly defined.2 The prevalence rate of lymphedema in women treated for breast cancer has been reported as 11%3 and 25%.4 Lymphedema is not well understood as the mechanisms responsible for normal lymph flow are still unclear. Despite the importance of the lymphatic system in fluid and macromolecule exchange, our understanding of it lags far behind that of the vascular system, partly because it is difficult to study such tiny and thin vessels.
Lymph vessels are found in all tissues save those with a low level of exchanges, as bone and cartilage and the particular case of the central nervous system. The lymphatic system plays an important role in lipid absorption as in the intestinal tract where it is particularly developed. It is also involved in immune reactions. Lymph vessels carry immune factors and cells (lymphocytes) to the tissues and lymph nodes, and act as filters and reservoirs for white blood cells and tumor cells.5 However, the most important function of lymph vessels is to maintain fluid and macromolecule balance and oncotic pressure. Plasma filters into the interstitial space from blood through the capillaries. Much is reabsorbed by tissue cells or blood, but not all because of osmotic forces resulting from protein extravasation. Lymph vessels drain this excess fluid to venous blood to avoid edema. Proteins that leak out of capillaries into the interstitial space return to the blood through the permeable lymph vessels, thus ensuring homeostasis. Otherwise, if the blood osmotic pressure falls, fluid imbalance and its consequences will ensue. Because of the role of lymph vessels, lymphedema is often associated with venous disease and cancer. Fluid accumulation in tissues also causes fibrosis, chronic inflammation, and tissue changes.
The lymphatic system can be considered as an organ in its own right, as recent research work has demonstrated lymphangiogenic factors, specific lymphatic markers, and lymphatic endothelial cells (which differ from vascular endothelial cells). The idea of a specific organ is confirmed by the fact that lymph sacs are already present in 6- to 7-week-old human embryos, sprouting from embryonic veins.6
PHYSIOLOGY OF THE LYMPHATIC SYSTEM
Functional anatomy
The lymphatic system is composed of capillaries, collecting vessels, lymph nodes, trunks and ducts, each part with a specific anatomy and role. Lymph vessels can anatomically be divided in 2 parts, initial lymphatics and collecting lymphatics. The initial lymphatics are located in tissues close to blood microvessels. Even intravital microscopy, which reveals microvessels and red blood cells, cannot distinguish initial lymphatics, whose size (10 µm to 60 µm diameter) has hampered investigations. By injection of microspheres into the arterioles, combined with histological techniques, Schmid-Schönbein7 has recently found that the initial lymphatics have a wall of loose, flattened, overlapping endothelial cells that are depleted in adhesion molecules as VE-catherins. Anchoring filaments bind the endothelial cells tightly to surrounding tissues. The discontinuity of the basal lamina allows macromolecules and cells to reach the lymph.8 Schmid- Schönbein suggested that the initial lymphatics contain endothelial microvalves (Figure 1),9 which allow the fluid to enter but not leave the interstitium.7 Initial lymphatics are not contractile, but lymph formation in them requires periodic expansion and compression of surrounding tissues.
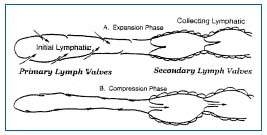
Figure 1. Two-valve system in lymphatics: primary valve in the initial lymphatic and secondary valve in the collecting lymphatic.
During expansion, the interstitial fluid can enter the lymphatics through the endothelial microvalves, because the intralymphatic pressure is lower than the interstitial fluid pressure. Compression of surrounding tissues forces the lymph towards the collecting lymphatics, whose smooth muscle can spontaneously contract. The valve-containing part of a lymph vessel and the adjacent portion of the vessel before the next valve form a functional unit called the lymphangion, which is able to contract or expand. Lymphangions are clearly seen by intravital microscopy of the rat mesentery circulation, close to the microvascular system and around lipid cells (Figure 2). They show spontaneous contractions and their valves can be easily seen in Figure 3.
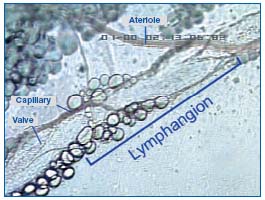
Figure 2. A lymphangion of rat mesenteric lymphatics seen by intravital microscopy.
Figure 3. A valve (opened and closed) of rat mesenteric lymphatics seen by intravital icroscopy.
Collecting lymphatics drain initial lymphatics toward the nodes. Pre- and postnodal lymphatics can be distinguished. Lymph nodes crossed by collecting lymphatics are organized in clusters and play an important part in the exchange between lymph and blood. White blood cells, but also tumor cells, may proliferate in lymph nodes and reach the blood and organs. White blood cells in the nodes phagocytose proteins and then eliminate them from lymph.
Collecting lymphatics widen into trunks that enter the thoracic ducts where the lymph returns to the blood stream. Right lymphatic ducts receive lymph from the right upper quadrant and drain into the right subclavian vein. Apart from this, all the lymph drains into the thoracic duct, which drains into the left internal jugular vein. The exceptions are the intestinal, hepatic and lumbar lymphatics, which drain to the cisterna chyli in the abdominal cavity.
As the lymph vessels are not replete with fluid, they are protected from hydrostatic problems during gravitational stress, unlike veins. The lymphatic system includes lymph (circulating fluid), vessels (parallel to veins), nodes along collecting vessels, and isolated nodules in the intestinal wall and specialized organs (as tonsils, thymus, and spleen). Unlike the vascular system, the lymphatic system is not a closed circuit.
Pump activity
The lymphatic system has all the anatomic components required for active pumping of the interstitial fluid. For years, the lymphatic system was considered as a passive pump and excited limited interest. In recent decades, studies have demonstrated the pumping activity of the lymphatics. To work, a pump needs a pressure and volume difference, and this can be generated by the contractility of the vessels or by changes in external pressure. The initial lymphatics have two valve systems: endothelial microvalves and classical intralymphatic valves. This twovalve system provides a mechanism for unidirectional flow during compression and expansion of the initial lymphatics. These compression and expansion movements depend on muscle contraction, breathing movements (particularly inspiration), arterial pulsations, postural changes, and skin tension.10 Lymph flows at around 125 mL/h, and this rate may be increased 10- fold during exercise.
Lymphangions can act as a pump when actively transporting lymph against a pressure gradient, or as a conduit when passively transporting lymph down a pressure gradient as described by Quick11 and Gashev.12 These authors compare the lymphangion to a ventricle. Transmural pressure is an important hydrodynamic factor for the contractility of lymphangions. It modulates the strength and frequency of contractions. Zhang et al13 described a motion wave propagation from one lymphangion to the next due to a pacemaker site at the inlet side in the valve in each lymphangion. The flow is related to the pressure changes between two lymphangions and stimulates a new contraction. Quick and Gashev suggest that the response of endothelium to wall tension and shear stress close to the inlet valve site could be one of the mechanisms responsible for lymphatic motion.
The conducting lymphatics contain smooth muscle that contracts at a rate of 1 to 15 cycles per minute,14 in phase opposition: one lymphangion contracts when the next dilates, so one is empty when the next is full.
Lymphatics have a low internal pressure (several mm Hg), but are very sensitive to pressure gradient, which has an influence in edema, for example. Other factors may interfere with pump activity. For example, the pump is only active at low oxygen tension (25-40 mmHg). Conversely, a high level of oxygen inhibits the frequency and amplitude of the contractions.
The lymphatic system is active, but some authors suggest that whether it is active or passive depends on the environment. In some diseases, where a substantial fluid drainage is needed, the lymphatics dilate and lose their contractile activity. Using mathematical models, Quick et al11 have demonstrated a pressure gradient along lymphatics with an excess pressure in the surrounding tissues. In this case, drainage is better if the vessels are dilated rather than spontaneously contracting.
The lymphatic system is highly adaptable, and sensitive to small internal or external pressure differences. Embryologically, lymphatic endothelial cells can be distinguished from vascular endothelial cells, and have specific receptors, suggesting they also have a specific pharmacological role.
PHARMACOLOGY OF THE LYMPHATIC SYSTEM
As the lymphatic system was compared to the vascular system, vasoactive drugs have been tested on lymphatics, but we know that receptors on lymphatic endothelial cells differ from those on vascular endothelial cells. Some vasoactive agents can regulate the activity of the lymphatics. Several agents, particularly cardiovascular drugs, have been tested in vitro (bovine isolated lymphatics) and in animals (sheep, rat). It was shown that lymphangions are sensitive to vasoactive drugs as nitric oxide (NO) donors,15,16 prostaglandins, and thromboxane.17 Vasodilators as NO tend to diminish the force and frequency of lymphatic pumping. Vasoconstrictors, as thromboxane, seem to have the opposite effect.
Using intravital microscopy on rat mesenteric lymphatics, adrenergic drugs were tested on lymphatic activity. The receptor antagonists alpha 1 (prazosin) and alpha 2 (yohimbine) do not modify the diameter or contractile activity of lymphatics, suggesting that there is no adrenergic tone in lymphatics. Norepinephrine and phenylephrine increase the frequency of contractions and decrease the diameter. These experiments indicate that lymphatic function can be increased through alpha- 1 but not alpha-2 adrenoreceptors.18
Bradykinin increases the frequency, strength, and duration of contractions of initial lymphatics.19,20 Its effect on mesenteric lymph vessels of the rat can be visualized using intravital microscopy (Figure 4).
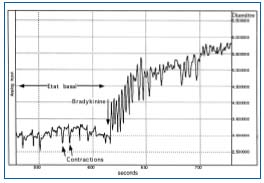
Figure 4. Effect of bradykinin on lymphatic contractions.
Against the backdrop of the recent interest in lymphatics and the demonstration that the lymphatic system is a system apart and different from the vascular system, with specific lymphatic endothelial cells, Ohhashi21 has reviewed new pharmacological research on lymphatics. For example, the generation of endogenous NO and reactive oxygen radicals (ROR) from lymphatic endothelial cells, and the activation of ATP-sensitive potassium channels (KATP), were shown to play a role in the regulation of lymph transport. NO released from these endothelial cells (through the constitutive NO synthase) inhibits rhythm and amplitude of the pump activity. ATP induces dilation and also inhibits lymphatic pump activity. Acetylcholine provokes relaxation of the lymph vessels through the release of NO and has negative chronotropic and inotropic effects on the pump. Endothelin increases lymphatic vasomotion involving calcium.
Because of the role of lymphatics in tumor metastases, Ohhashi also mentions substances released by tumor cells, as NO and derivatives, which reduce pump activity. Exuded macrophages present in the lymph vessels or nodes, when they are activated by bacterial lipopolysaccharides, release NO and vasodilating prostaglandins, thereby decreasing pump activity. Edema is one of the consequences of low pump activity.
LYMPHATIC SYSTEM IN DISEASE
Damage to the lymphatic system can cause lymphedema. Primary lymphedema is an inherited condition, which can appear in different parts of the body and at different ages. More common is secondary lymphedema, which can be due to inflammation, invasion of bacteria or parasites, occlusion after surgery, or irradiation of tumors. This is the clinical manifestation of an imbalance of forces at the capillary wall. Edema is an excess accumulation in the interstitial space of fluid that has not been reabsorbed by capillaries or taken up by lymphatics. It can occur because of obstructions, lymphatic insufficiency, increased protein permeability, inflammation, and reduction in plasma proteins. In humans, benzopyrones (coumarin, oxerutins, and diosmin), flavonoids, and ruscus extract are used to treat high-protein edema. They reduce swelling and pain, and improve healing and oxygenation. Benzopyrones increase the number of macrophages, which lyse excess proteins, and improve pumping by collecting lymphatics.22
The lymphatic system has a major role in immune defense.23 The lymph vessels and nodes transport antibodies, lymphocytes, but also bacteria. The lymphatic system plays a major role in all diseases involving an inflammatory process (rheumatoid arthritis, lupus, scleroderma). In AIDS, HIV can be propagated through the lymph vessels, which could be a target for antiviral medication. The lymphatic system also interacts with digestion, helping reabsorption of fat, and its dysfunction can lead to malnutrition, ascites, and obesity.
As seen above, the lymphatic system is of importance in many diseases of different organs (liver, heart, kidney, stomach, blood) and different causes (virus, bacteria, hemorrhagic shock, organ transplantation, autoimmunity). In most cases, the clinical sign is edema.
ROLE OF THE LYMPHATIC SYSTEM IN SPREAD OF CANCER
The lymphatics play a crucial role in the dissemination of solid tumors, particularly of the breast, lung, colon, and prostate.5 Tumor cells are transported through lymph vessels into lymph nodes and are then disseminated to other nodes and organs. Tumor cells themselves induce lymphangiogenesis by secreting substances that trigger the proliferation of lymph vessels. The lymphatic system itself may also be the site of cancer, such as lymphoma, which is due to the transformation of lymphocytes.
CONCLUSION
Following years during which the lymphatic system was neglected, new work has shown that it plays a central role or is implicated in inflammation, cancer, asthma, transplant rejection, and lymphedema. This renewed research interest has already led to advances in prevention and treatment, such as an anti-VEGFR-3 antibody, which inhibits lymphatic regeneration, and gene or gene-product therapy of lymphedema.24
REFERENCES
2. Williams AF, Franks PJ, Moffatt CJ. Lymphoedema: estimating the size of the problem. Palliat Med. 2005;19:300-313.
3. Edwards T. Prevalence and aetiology of lymphoedema after breast cancer treatment in southern Tasmania. Aust N Z J Surg. 2000;70:412-418.
4. Logan V. Incidence and prevalence of lymphoedema: a literature review. J Clin Nurs. 1995;4:213-219.
5. Swartz MA. The physiology of the lymphatic system. Adv Drug Del Rev. 2001;50:3-20.
6. Alitalo K, Tammela T, Petrova TV. Lymphangiogenesis in development and human disease. Nature. 2005;438:946-953.
7. Trzewik J, Mallipatu SK, Artmann GM, et al. Evidence for a second valve system in lymphatics: endothelial microvalves. FASEB J. 2001;15:1711-1717.
8. 0’Morchoe CC, 0’Morchoe PJ. Differences in lymphatic and blood capillary permeability: ultrastructural-functional correlations. Lymphology. 1987;20:205-209.
9. Mendoza E, Schmid-Schönbein GW. A model for the mechanics of primary lymphatic valves and the interaction with the secondary valves. Paper presented at Summer Bioengineering Conference; June 25-29, 2003; Key Biscayne, Florida, USA.
10. Schmid-Schönbein GW. Microlymphatics and lymph flow. Physiol. Rev. 1990;70:987- 1028.
11. Quick C, Venugopal AM, Gashev AA, et al. Intrinsic pump-conduit behavior of lymphangions. Am J Physiol Regul Integr Comp Physiol. In press.
12. Gashev AA. Physiologic aspects of lymphatic contractile function. Ann N Y Acad Sci. 2002;979:178-187.
13. Zhang J, Li H, Xiu R. The role of microlymphatic valve in the propagation of spontaneous rhythmical lymphatic motion in rat. Clin Hemorheol Microcirc. 2000;23:349-353.
14. McHale NG, Meharg MK. Co-ordination of pumping in isolated bovine lymphatic vessels. J Physiol. 1992;450:513-512.
15. Shirasawa Y, Ikomi F, Ohhashi T. Physiological roles of endogenous nitric oxide in lymphatic pump activity of rat mesentery in vivo. Am J Physiol Gastrointest Liver Physiol. 2000;278:G551-G556.
16. Hagendoorn J, Padera TP, Fukumura D, Jain RK. Molecular regulation of microlymphatic formation and function: role of nitric oxide. Trends Cardiovasc Med. 2005;15:169-173.
17. Johnston MG, Gordon JL. Regulation of lymphatic contractility by arachidonate metabolites. Nature. 1981;293:294-297.
18. Benoit JN. Effects of alpha-adrenergic stimuli on mesenteric collecting lymphatics in the rat. Am J Physiol Regul Integr Comp Physiol. 1997;273:R331-R336.
19. Unthank JL, Hogan RD. The effect of vasoactive agents on the contractions of the initial lymphatics of the bat’s wing. Blood Vessels. 1987;24:31-34.
20. Yokoyama S, Benoit JN. Effects of bradykinin on lymphatic pumping in rat mesentery. Am J Physiol Gastrointest Liver Physiol. 1996;270:G752-G756.
21. Ohhashi T, Mizuno R, Ikomi F, Kawai Y. Current topics of physiology and pharmacology in the lymphatic system. Pharmacol Ther. 2005;105:165-188.
22. Casley-Smith JR. The benzo-pyrone drugs in the treatment of lymphedema (and other protein edemas). Lymphedema Association of Australia, 1998. Available at: http://www.lymphedema.org.au/bp.html.
23. Burns R. Importance of the lymphatic system. The Lymphatic Research Foundation. Available at: http://www.lymphaticresearch.org.
24. Rockson SG. Therapeutics for lymphatic disease: the role of the pharmaceutical and biotechnology sector. Lymphatic Resaearch and Biology. 2005;3:103-104.