Modeling and 3D printing of veins from CT venograms
of the UNESCO Chair of Digital Anatomy,
Paris University
Abstract
Digital anatomy has more and more applications in medicine and surgery, thanks to the progress in imaging and power of computer software. To evaluate patients with chronic venous disorders, in the case of complex anatomy or recurrent varices after surgery (REVAS), three-dimensional (3D) modeling of the venous system is often a great support. A global 3D depiction of the whole venous morphology will help the hemodynamical mapping achieved by color Duplex ultrasound. In addition to anatomical information, color Duplex ultrasound also provides essential hemodynamic data for the treatment of each particular patient. This paper explains how to build and print 3D models of the veins. Data are provided by computed tomographic (CT) venography. The 3D reconstruction is possible through use of three software freely available on the internet: Horos® (Mac computers only), Meshmixer®, and Cura®. The resulting 3D models are easily displayed and handled on a personal computer, tablet, or smartphone and could be shared within 3D-model communities on the web. In the field of anatomy and in surgical simulations, 3D modeling of the human body is revolutionary. The primary aim of the UNESCO Chair of Digital Anatomy (Paris University) is the dissemination and sharing of digital tools and models for educational anatomy (www.anatomieunesco.org).
Introduction
Venous anatomy is complex and highly variable. For this reason, before any decision or treatment, a complete check of venous morphology and hemodynamics should be done via mapping in all patients with chronic venous disorders (CVD).
Color Duplex ultrasound is an evaluation method that may be used daily and reliably for building a venous map of patients, always performed while the patient is in the standing position. But in some cases, more detailed information about the venous anatomy of the whole network is needed, in particular, for deep veins. Moreover, this in-depth investigation of the deep system could discover some abnormality1 or anatomical variation that could be a cause of so-called “primary” CVD. In fact, we call it primary in most cases because we do not find any cause.
Materials and methods
The technique of investigation and indications for computed tomographic (CT) venography are described with more detail in our previous publications.2-5 Here, we provide a brief summary of the CT venography protocols (Table I) and the indications for CT venography for patients with CVD.
CT venography could be used for education and research, but in most cases, the aim is venous assessment of patients with CVD.
The result of CT venography is a set of axial slices in DICOM® format (Digital Imaging and Communications in Medicine standards). The DICOM is the international standard of medical imaging, universally used by radiologists and practitioners for diagnostic purposes in radiology all over the world.6 It contains both an image and a collection of data about the exam and the patient.
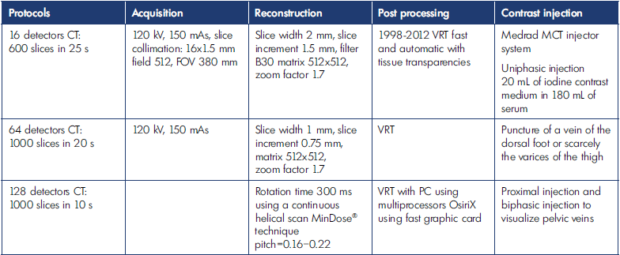
CT, computed tomography; FOV, field of view; VRT, volume rendering technique; MDCT, multidetector computed tomography; MCT, multislice CT (MSCT)
Table I. Multislice spiral computed tomography (CT) protocols for CT venography.
This file format could be visualized and manipulated by dedicated software called DICOM® browsers (Table II).
Indications2 for CT venography are mainly patient assessment and anatomical studies useful for research and for learning of anatomy, as well as for use by surgeons to run a preoperative simulation or for planning (Figure 1).
3D models are reconstructed from DICOM slices produced by CT venography. Models are built in three steps, through use of the following available free software:
• Horos®7 (only for Mac computers) is a DICOM® browser and provides a 3D reconstruction of the venous anatomy. It produces 3D vector models, also called 3D mesh, obtained by a segmentation process.
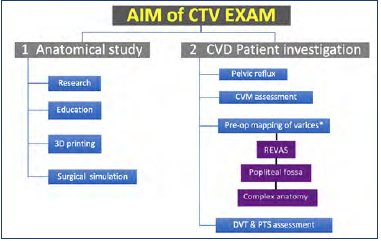
Figure 1. Indications of computed tomography (CT)
venography.2
1) CT venography allows 3D interactive virtual dissection of
the limb for education or simulation.
2) CT venography provides pure morphological information;
therefore, Doppler ultrasound is mandatory for hemodynamical
assessment. *Particularly for recurrent varices after surgery
(REVAS), complex cases, and popliteal fossa recurrence.
Abbreviations: 3D, three-dimensional; CTV, computed
tomographic venography; CVD, chronic venous disease;
CVM, congenital venous malformations; DVT, deep-vein
thrombosis; PTS, post-thrombotic syndrome.
• Meshmixer®8 is then used to clean, simplify, and repair the huge 3D mesh file produced by Horos®.
• Cura®9 is finally used to build a “gcode” file. This will tell the 3D printer how to slice and print the 3D anatomical model.
Horos®
Two types of 3D reconstruction could be created by Horos® from the DICOM digital data as follows: i) volume rendering (VRT); and ii) surface rendering, also called vectorial modeling. In both cases, the main process is the “segmentation” of the anatomical data:
“Segmentation” means to outline and draw the boundaries of each anatomical structure. Each anatomical element (bone, skin, muscle, fat tissue) is automatically identified by its level of density (4096 levels of Hounsfield units in DICOM® slices). This operation on the image is named thresholding, which consists of a selection of a sample of densities that eliminates others. By this technique, one can erase some specific anatomical structures or make them transparent.
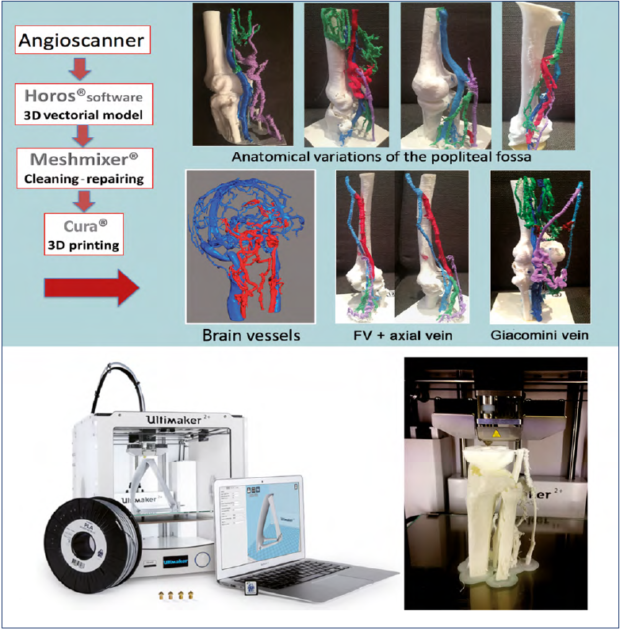
Figure 2. Methodology of 3D printing of educational models of venous anatomy. Workshop presented at Charing Cross 2019
and Krakow’s 2019 International Union of Phlebology (UIP) Chapter meetings.
Abbreviation: FV, femoral vein.
Practical use of Horos®
After opening the list of the DICOM® exams, click to display the patient’s file. This opens the 2D window showing the slices. Then choose a reconstruction protocol by clicking on the gray wheel located on the toolbar and selecting one of the seven 3D protocols listed in the menu (Figure 3). These seven are multiplanar reconstruction (MPR), curved MPR, orthogonal MPR, maximum intensity projection (MIP), volume rendering (VRT), surface rendering (SR), and virtual endoscopy.
The protocol for easy building of 3D vectorial models is 3D surface rendering. For this, we set up the parameters to obtain different segmentations of the tissues (Figure 4). In most cases, the pixel value will be 100 and resolution, 90% to obtain a good segmentation of the bone and injected vessels. These parameters could be refined as necessary. The skin and lungs could also be segmented during the same process by choosing a second structure and choosing -300 as the pixel value of the second surface.
The huge 3D vectorial “mesh” model that is thus obtained has to be exported into “obj” or “STL” format. This could be done using the export menu (gray wheel on the toolbar).
Meshmixer®
We first open the obj file exported by Horos® Manipulation of the mesh model is controlled through the right mouse button (3D move), through dragging the mouse wheel down (translation), and through wheel roll (zoom). The left mouse button is for selection of objects or menu options.
The aim of Meshmixer® is to clean the file by erasing the small isolated pieces, and to perform a further segmentation of its anatomical structures, mainly veins and bones. We use the following functions of Meshmixer_ directly available through the following function keys: i) E extends the selection to all connected points; ii) Y separates the selection and creates a new layer; iii) X erases the selection; and iv) I inverts the selection. Several other functions are available, including color painting of the objects, sculpting the objects (inflate, smooth, flatten, etc) with brushes, and plane cutting to divide the mesh.
The main issue for segmentation is to separate the different structures by erasing their mesh connections. Further colorization of each anatomical element is possible to better visualize the 3D morphology and display animations.
The other main interest of Meshmixer® is to repair the 3D mesh and arrange it to be printable. The menu option “analysis” shows and repairs the holes, missing parts, or defects that could be removed for a better result of the 3D printed object.
The 3D file is then exported in obj format to be printed with Cura®.
Cura®
The aim of Cura® software is to divide the 3D anatomical mesh and compute it into thin slices to be added by the printer’s head one by one onto the horizontal plate.
A number of parameters have to be set up according to the printer model, time, resolution, and quality of the printed model obtained.
We regularly organize courses and workshops with our partners to promote these new educational tools through the UNESCO Chair of Digital Anatomy5 (Paris University). The goal is to learn more about the practical use of these software in order to produce 3D anatomical models. Please visit our website for more information: www. anatomieunesco.org.
An educational video is also available on our YouTube TV channel at https://youtu.be/JmJ3yLcTUS0.
Results
• CT venography is both a great educational tool for learning venous anatomy and a powerful research tool for improving our understanding of the venous system.
• Through Horos® 3D animations, rotational models can be built and “journeys” taken inside the body.
• 3D modeling allows virtual dissection of the limb; it is a powerful teaching and learning tool for students of human anatomy in order to prepare for, not replace, cadaver dissection (see table of virtual dissection in reference 10; see Figure 5).
• 3D printing of anatomical models is a great tool to study anatomical variations, which are common in the venous network.
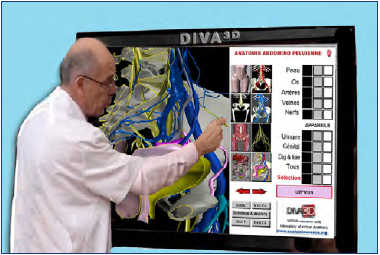
Figure 5. Virtual dissection table10 (Diva3D). The lifesize
anatomical model could be manipulated on a big
touchscreen with only three fingers.
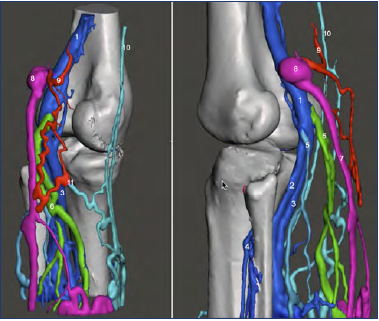
Figure 6. Colored vectorial 3D model (Meshmixer) showing
an aneurism of the saphenopopliteal junction (SPJ).
1, popliteal vein (PV) in dark blue; 2, lateral root of the PV;
3, medial root of the PV; 4, anterior tibial veins; 5, lateral
gastrocnemial veins (in blue); 6, medial gastrocnemial veins
(in green); 7, small saphenous vein (SSV) in purple; 8, aneurism
of the SPJ; 9, thigh extension of the SSV (in red); 10, great
saphenous vein (GSV; light blue); 11, oblique communicating
vein.
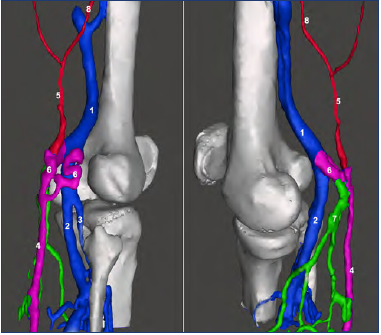
Figure 7. Colored vectorial 3D model (Meshmixer) showing
a duplicated termination of the small saphenous vein (SSV).
1, popliteal vein (PV) in dark blue; 2, medial root of the PV; 3,
lateral root of the PV; 4, SSV (in pink); 5, thigh extension of the
SSV (in red); 6, ring-shaped termination of the SSV; 7, medial
gastrocnemial veins (in green); 8, thigh perforator.
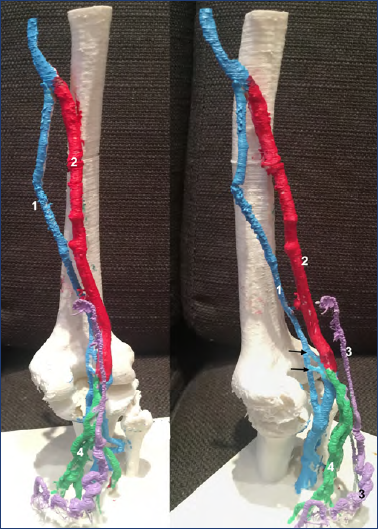
Figure 8. 3D printed model of a femoral vein duplication.
1, femoral vein in the Hunter canal (in blue); 2, axial vein
along the sciatic nerve (in red); 3, small saphenous vein (SSV;
in purple) dystrophic and dilated; 4, medial gastrocnemial
veins (in green).
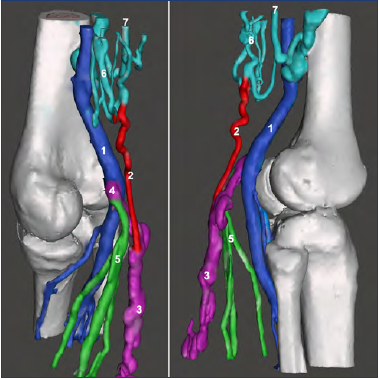
Figure 9. Colored vectorial 3D model (Meshmixer) showing
a common trunk between the small saphenous vein (SSV) and
the medial gastrocnemial vein (GV) and a thigh extension of
the SSV.
1, popliteal vein (PV) in dark blue; 2, thigh extension of SSV
(in red); 3, small saphenous vein (SSV) in purple; 4, common
trunk of the SSV with the medial GV (in purple); 5, medial GV
veins (in green); 6, deep femoral vein; 7, venous arcades of the
semimembranosus muscle.
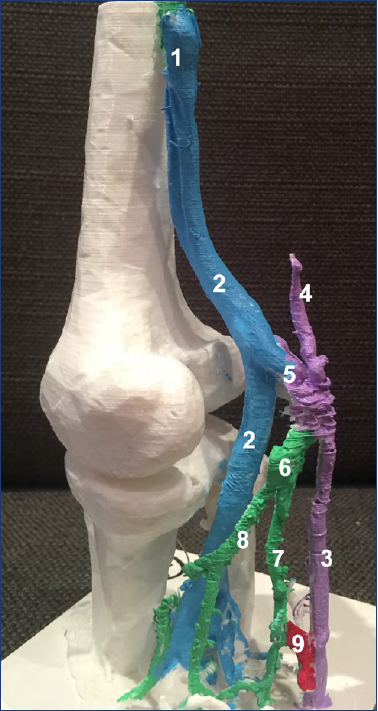
Figure 10. 3D printed model of a common trunk between the
small saphenous vein (SSV) and the medial gastrocnemial vein
(GV).
1, femoral vein (in blue); 2, popliteal vein (in blue); 3, SSV
(in purple); 4, thigh extension of SSV (in purple); 5, common
trunk SSV-GV; 6, trunk of medial GV (in green); 7, dorsolateral
component of the medial GV; 8, ventromedial component of
the MGV; 9, perforating vein of the calf (in red).
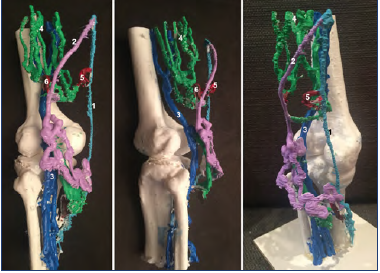
Figure 11. 3D printed model of a Giacomini vein.
From left to right posterior, lateral, and medial views.
1, great saphenous vein (GSV; in light blue); 2, Giacomini vein
(in purple); 3, popliteal vein (in dark blue); 4, venous arcades
of the semimembranous muscle (in green); 5, Hunterian
perforator vein with GSV (in red); 6, thigh perforator with the
Giacomini vein.
Examples of variations of the small saphenous vein (SSV) termination and the femoral vein variations are shown in Figures 6 to 12.
For sharing 3D anatomical models, several websites are available. Some of them are totally free, and you can even download several printable 3D models. These sites include i) www.embodi3d.com (biomedical 3D printing), with the possibility to automatically convert CT scans into 3D printable models for free with democratiz3D®; and ii) NIH 3Dmodels.com, which has a large collection of 3D models of vascular cardiac pathology.
Other web solutions propose to host your models; for example, Sketchfab® (www.sketchfab.com).11 With such solutions, you subscribe to buy or sell your own collection of 3D models. You can include labels of the structures (Figure 13) and display the model in virtual reality (VR) mode (Figure 14).
Another interesting possibility is to display your own models on your tablet or smartphone (Figure 15). This is possible with the free software named 3D PDF reader, available on the App Store or Google Play. The only limitation is the size of your 3D model (may not exceed 15 000 faces).
Using these different tools, educational anatomy is now entering a new era in which these 3D models are available for everyone willing to teach or learn human anatomy. They could also be used together with an e-learning platform like we do on the website of the UNESCO Chair of Digital Anatomy (www.anatomieunesco.org).
The main example is the Visible Patient® software created by IRCAD (Research Institute Against Digestive Cancer) (Figure 16).12 This makes possible the surgical use of 3D vascular models. Such models show the vascular segmentation of the main organs, which is necessary for making decisions and for good surgical planning. Its application would be useful in hepatic surgery (Figure 17), kidney surgery, lung surgery, orthopedics, dental implantology, and in neurosurgery.
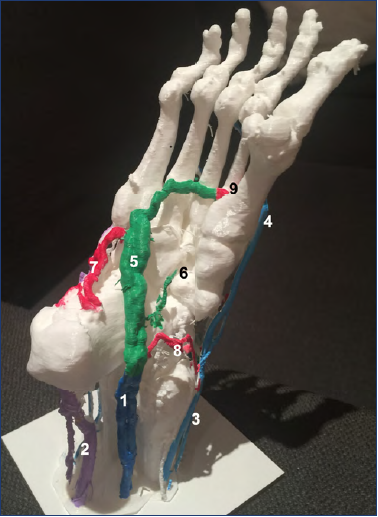
Figure 12. 3D printed model of the foot veins.
Medial view of the sole.
1, posterior tibial veins at the calcaneus convergence (in blue);
2, small saphenous vein (SSV; in purple, dilated); 3, great
saphenous vein (GSV; in blue); 4, medial marginal vein; 5,
lateral plantar veins (foot pump, in green); 6, medial plantar
veins; 7, calcaneus perforator vein (in red); 8, inframalleolar
perforator vein (PV); 9, PV of the first metatarsal interspace.
The aim of these 3D anatomical models of the patients before surgery is to have an in-depth knowledge of the vascular anatomy. This makes it possible to simulate the operation according to the personal anatomy of the patient and the organ segmentation.
This makes possible the new world of image-guided surgery: mini-invasive, more controlled, more accurate, and safer because it avoids the main complications.
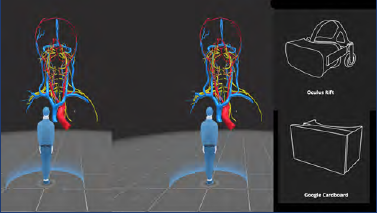
Figure 14. Display of the 3D model in virtual reality (VR) mode
with labels via Sketchfab.
You can visualize the model in stereovision with your
smartphone inserted into a cardboard or an Oculus mask.
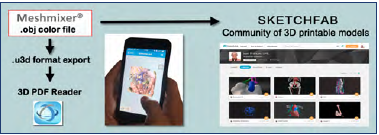
Figure 15. Use of educational models of anatomy on tablets,
smartphones, and web communities of models.
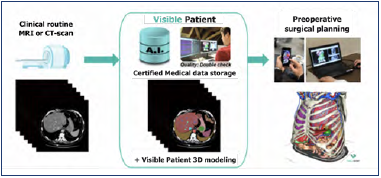
Figure 16. Visible Patient software™ is a company resulting
from 15 years of research by the IRCAD R&D department
in computer-assisted surgery. Visible Patient proposes a
connected solution providing a 3D model of a patient from
his/her medical image sent through a secured internet
connection.
Abbreviations: 3D, three dimensional; AI, artificial intelligence;
CT, computed tomography; MRI, magnetic resonance imaging.
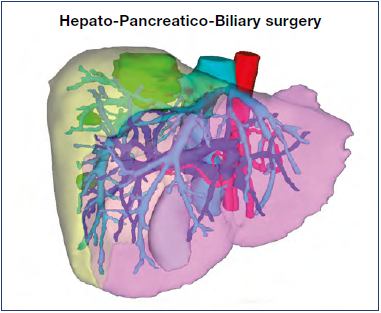
Figure 17. Visible Patient software: planning for hepatic
surgery.12
(By permission from Professor Luc Soler, IRCAD – Strasbourg.)
The 3D modeling of the vessels of the liver and of the
segmentation makes it possible to make decisions about the
type of excision (segmentectomy, partial hepatectomy) and the
technique to be followed. Here, several liver metastases are all
located in segment VIII.
Conclusion
The new tools of 3D modeling are revolutionary for educational anatomy and for clinical applications in the case of complex venous anatomy. It is also the future of surgery, providing accurate information about the vascular anatomy of each particular patient. Modern surgery has to be image-guided surgery for elective and more limited ablation of organs (segmentectomy).
REFERENCES
1. Gaweesh AS, Kayed MH, Gaweesh TY, Shalhoub J, Davies AH, Khamis HM. Underlying deep venous abnormalities in patients with unilateral CVD. Phlebology. 2013;28:426-431.
2. Uhl JF. Three-dimensional modelling of the venous system by direct multislice helical computed tomography venography: technique, indications and results. Phlebology. 2012;27:270-288. doi:10.1258/phleb.2012.012J07.
3. Uhl JF, Caggiati A. Multidetector-row CT angiography: 3D evaluation of the venous system in varicose limbs by multidetector spiral CT. In: Catalano C, Pasariello R, eds. Medical Radiology – Diagnostic Imaging. Springer; 2005:199- 206. Available at: http://link.springer. com/10.1007/3-540-26984-3_15/.
4. Uhl JF, Verdeille S, Martin-Bouyer Y. Three-dimensional spiral CT venography for the pre-operative assessment of varicose patients. VASA. 2003;32:91-94.
5. Uhl JF, Ordureau S. The new computer tools of virtual dissection to study anatomy of the vascular system. Phlebolymphology. 2008;15:151-155.
6. Digital Imaging and Communications in Medicine. The DICOM Standard. Available at: http://dicom.nema.org/ standard.html.
7. Horos [software]. Version 4.0. Horos Project. Available at: https:// fr.freedownloadmanager.org/Mac-OS/ Horos-GRATUIT.html.
8. Meshmixer [shareware]. Version 3.5. Updatestar. Available at: https:// meshmixer.updatestar.com/fr.
9. Cura® [software]. Version 3.6.0. Updatestar. Available at: https://cura. updatestar.com/fr.
10. Digital Anatomy: Applications of Virtual, Mixed and Augmented Reality. Uhl JF, Jorge J, Lopes DS, Campos PF, eds. Switzerland: Springer Nature; 2021.
11. Sketchfab® website. Available at: https://sketchfab.com.
12. IRCAD France. Visible Patient [software]. Available at: www.ircad.fr/research/ visible-patient.