Recent findings in the pathogenesis of venous wall degradation
Université Victor Segalen Bordeaux 2
Bordeaux, France
SUMMARY
Increased venous pressure in the erect position is a constant finding in patients with chronic venous disease. In the case of primary chronic venous disease, this increase in venous pressure is linked to venous reflux. A linear relationship exists between increased ambulatory venous pressure and skin ulceration.
The length of time a subject is exposed to elevated venous pressure is a major factor in the progression of venous disease and in the development of varicose veins. The development of an animal model of venous hypertension (VHT) has demonstrated venous valve damage at an early stage of the disease, associated with the development of a leukocyte-endothelium biochemical cascade reaction. According to a similar mechanism, this inflammatory chain reaction may extend to large veins, resulting in multilevel valvular deficiencies and increased VHT. Prolonged VHT has an impact on the capillary network, and can produce trophic (skin) changes. Factors that trigger leukocyte adhesion and migration in the venous endothelium are mainly related to slowing of blood flow, with a decrease in shear stress in the valvular sinuses and along the large veins, and lastly in the cutaneous venules. Valvular and subsequent luminal and medial hypoxia is also a triggering factor. Remodeling of the saphenous veins is related to migration of monocytes, T and B lymphocytes, and mast cells in particular. The latter transport growth factors such as transforming growth factor (TGF_1). All these cells, including the endothelium and migratory and dedifferentiated smooth muscle cells, participate in setting up enzyme systems that act on the extracellular matrix (ECM), in particular zinc-dependent metalloproteinases (MMPs), which are produced in excess compared with their inhibitors. Collagen disorders (type III rather than type I), rarefaction of elastin, alternating cellular zones, and atrophic fibrous zones account for the macroscopic and sometimes exuberant appearance of varices. The wide variability from one subject to another in the progression of venous disease is related to many factors that promote venous disease, ranging from having to stand erect to familial genetic factors.
In summary, alterations in venous valves induced by a leukocyte-mediated inflammatory process appear to be a key factor in the development of varicose veins, and can be inhibited by the micronized flavonoid fraction in MPFF at a dose of 500 mg.
INTRODUCTION
Many causes of chronic venous disease (CVD) can be differentiated, but it is formally established that the most frequent one, primary venous disease, is linked to venous hypertension (VHT). The mechanisms responsible for venous return are complex, all the more so in that the force of gravity in the blood column in the erect position, venous distensibility, and pressure produced by abdominal exertion inhibit the return of blood to the heart in a subject in the standing position. Reflux is prevented by the venous valves, which protect against excessive distension of the venous wall, by facilitating appropriate emptying of the superficial venous network into the deep veins, and also protect distal tissues, especially the cutaneous tissue, which behaves “naively” with regard to VHT. Therefore, it is evident that venous valve incompetence, whose consequence is an increase in venous pressure, is the major cause of varicose vein disease, and subsequent venous insufficiency and skin disorders, including venous ulceration, the most serious complication. The historic studies by Nicolaides demonstrated a linear relationship between ambulatory venous pressure, measured at the ankle, and ulceration. Skin lesions, observed in stages 5 and 6 of the clinical, etiological, anatomical and pathophysiological (CEAP) classification, are most often associated with both superficial and deep venous reflux.1 The mechanisms by which uncontrolled VHT produces venous wall dystrophy, remodeling that characterizes varices and microcirculatory skin alterations, have been partly elucidated.
Recent studies show that the link between valvular dysfunction and remodeling involves early activation of aseptic inflammation, which triggers cellular and enzymatic processes. The cellular processes are dynamic, related to VHT, and again it is the cells that determine in situ the biochemistry of the valvular apparatus and in the wall of the vein.2,3
These findings represent an appreciable step in the study of venous disease, and enable better definition of new therapeutic targets. Nevertheless, the wide variability in the expression of venous disease, the many factors that promote it, and the genetic factors that remain largely unknown, have opened the way to future research in this field.
The purpose of this article is to provide an updated account of our knowledge of the mechanisms involved in venous disease, by considering the venous valve and the venous wall, factors that promote this disease, and therapeutic prospects.
Background to the relationship between venous hypertension and valvular alterations
Incompetent venous valves
Spontaneous venous insufficiency does not exist in fourlegged animals, because venous pressure in their paws is low. In humans in the standing position, venous pressure measured at the ankle reaches 85 mm Hg; but ambulation produces a fall to 25 mm Hg. Therefore, frequent and regular walking by healthy subjects provides effective protection against the pathologic effect of the blood column pressure in orthostatic posture, as a result of the “joint and muscle pump.” This was true of our distant ancestors who had to walk long distances in search of food, and may still be true for a few isolated tribes of humans who live under primitive conditions. Since such conditions of life have disappeared, subjects who live under the conditions of “modern life” tend to remain standing or in the seated position longer, thus activating the muscle pump mechanism less. Consequently, they are subject to VHT for a longer time. It is the duration of the pressure exerted on the venous valves and venous wall that determines alterations, and is the essential factor in the development of CVD.
Figure 1 depicts the conditions under which venous valves operate. Venous valves in superficial, paratissular and perforating veins are closed when the subject is in the standing position, and during contraction of skeletal muscles, thus providing peripheral protection. These valves open when the deep venous system is emptied, creating a negative pressure gradient, and then they fill from the upper to the lower part of the vein, in particular emptying the microcirculatory plexuses of the skin. Thus, valvular incompetence in superficial veins is the key component of venous disease, and has been observed by angioscopy,4,5 showing retraction of the free borders, atrophy of the leaflets, and thickening of the opposite side of the wall. Furthermore, angioscopy has shown the frequency of alterations, sometimes in their early stages, in young subjects, with no marked alteration in the corresponding veins.4 This finding has been confirmed by epidemiological studies on very young subjects, showing reflux in an appreciable percentage of subjects, as shown by duplex scanning (the Bochum study).6 Thus, valvular incompetence may frequently occur prior to venous wall damage, and therefore would be a decisive factor in the progression of this disease.
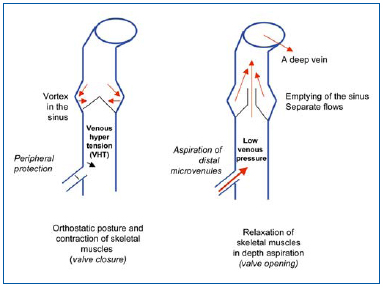
Figure 1. Alternating high and low venous pressures in the saphenous
veins in orthostatic posture. Occurrence of venous hypertension (VHT), specific to human subjects.
Figure 2 depicts hemodynamic alterations corresponding to valvular incompetence, with the occurrence of reverse flow prolonging VHT in the periphery, especially if the perforator veins are incompetent. The resultant hemodynamic disorders affect activation of the endothelium, a key player in triggering the inflammatory cascade.
It is undisputed that obstruction is a less frequent cause of venous ulceration than has been recognized to date, the cause being reflux in the superficial venous system, the perforator veins, or the deep venous system (mean values of several studies) in 80% of cases, while the sequelae of venous thrombosis or trauma are mentioned in 20% of cases, and a congenital anomaly is noted in 2%.7,8 CVD is maintained by an “axial reflux,” from the thigh to the calf, in the superficial and deep vein axes.
But then how does venous valve dysfunction produce histological alterations?
Several theories have been proposed, and currently two poles of thought have received a certain amount of acceptance: excessive distensibility in the venous wall, and constitutional weakness in the venous wall.
Because it contains few smooth muscle fibers, the venous wall is highly distensible. This property allows the vein to store large quantities of blood (concept of a venous reservoir). It has been suggested that repeated mechanical pressure by itself could produce abnormalities in the synthesis of collagen or elastin in the venous wall. An abnormal type of collagen has even been identified in cell cultures of fibroblasts from varices. Nevertheless, the link between mechanical and biochemical factors is missing, and increased distensibility appears to be secondary to remodeling. Constitutional weakness in the wall of the vein has also been suggested and points to involvement of hereditary factors. However, this notion applies little to the venous valve.
Ultrasonography studies have examined valvular functioning and have identified the direction and velocity of blood flow.
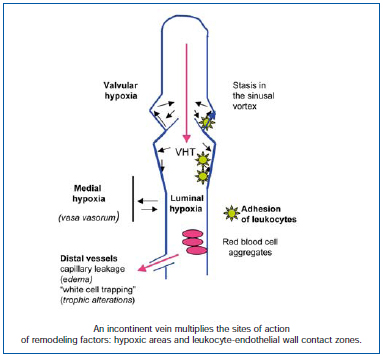
Figure 2. Incontinence of the valvular system produces areas of hypoxia: in the lumen of the blood vessel, by compression of the vasa vasorum, and at the bottom of the sinus. In addition, areas of leukocyte adhesion develop, as a result of lack of shear stress, early onset in the sinus, and along the altered blood vessel wall.
Progressive demonstration of the links between valvular outflow and leukocytes
Hemodynamic measurements in the sinus and during its cycle of action show sinuses as zones of branching flow, where the flow becomes organized into a vortex (Figure 3). The major part of the flow remains centripetal, while a small part reverses direction and fills the sinus in a counter-current direction. The residence time of circulating white blood cells therefore increases. Hamer et al have studied this type of outflow in dog veins made transparent by inducing a polystyrene microparticle flow that can be filmed. They described two types of vortex: a superficial vortex and a deep vortex.10 The superficial vortex is displaced towards the orifice of the sinus, while the particles make parabolic movements, slowly heading to the wall, promoting possible leukocyte adhesion. The vortex will be that much larger when the blood vessel is large. The deep vortex (the counterrotating secondary vortex) is usually empty, but under conditions of abnormal flow it fills in its capacity as a “cell trap.” Anoxia develops at the bottom of this vortex. Vortex size is kept small by the pulsatile flow, but increases if there is loss of pulsatility and venous stasis, creating conditions for leukocyte adhesion.10 In earlier studies, Sewitt observed platelets, red blood cells, and in particular leukocytes at the bottom of valves in deep veins using electron microscopy. According to Sewitt, venous thrombosis in valves in the deep vein system originate at a fibrinous platelet nidus, but he also noted that such a nidus is partially formed in normal persons, all the more so if they are elderly.11 Lurie defined the venous valve cycle, and noted a separation of flow at the time of an equilibrium phase (leaflets spread apart during systole), with the production of a vortex in the sinus crossed by slow currents (Figure 3). The dilated sinus is emptied by pulsatility, which allows reoxygenation of the valve bottom. Such functioning is multifactorial and all rheological factors and stasis alter it. Conversely, movements of the foot promote proper operation of the valvular apparatus (increased velocity, increased luminal pressure of the valve, as well as its potential for closure).12
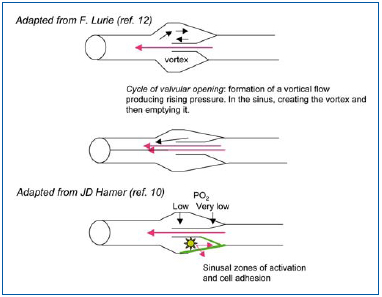
Figure 3. Examination of blood flow through the valvular system shows that vortices form in the sinus also under conditions of valve opening. Measurement of oxygen levels revealed hypoxia at the bottom. Adhesion molecules (VCAM-1, ICAM-1) developed on the anterior side of the cuspid and not below (see text and references).
All these studies have attracted the attention of hemorheologists, and, in particular, the San Diego team of Bergan, Takase, and Schmid-Shönbein, the latter being a founding member of the European Society of Hemorheology. These researchers understood that the conditions for outflow in the valve expose circulating white blood cells to hypoxia and shear stress. Hypoxia activates the endothelial cells, and the lowering of shear stress on the venous wall in contact with zones of slow flow allows leukocyte adhesion. Thus, a new concept to account for damage to the venous valve wall was developed.
Inflammation in the wall of the venous valve
Takase et al studied the course of valvular disease in an animal model by creating an arteriovenous fistula in the rat, according to a technique used by Van Bemmelen et al.13 After three weeks of elevated pressure, the valve leaflets were damaged, incompetent, retracted, and infiltrated by leukocytes. Identified by specific monoclonal antibodies (immunohistology), these white blood cells were granulocytes, and especially monocytes. In addition, T and B lymphocytes, and cells in apoptosis, were observed.14 Other experiments by the same authors based on occlusion of the rat mesenteric veins showed that adhesion of white blood cells was greater above rather than below the sinus. The opposite part of the venous wall underwent remodeling. In another study, Takase et al showed that the endothelial cells above the sinus expressed intercellular adhesion molecule-1 (ICAM-1).15 Thus the concept of the role of leukocytes in producing valvular damage was demonstrated. Moreover, it should be kept in mind that other authors had shown that rats trained to remain on their hind legs (vertical position) for several weeks (the so-called “standing rat” technique) showed venous valve remodeling.16
In humans, leukocytes were observed in venous valves in varicose vein disease, in particular monocytes17 and mast cells.18 Furthermore, the San Diego team demonstrated that, in venous insufficiency, “plasma inflammation” can be observed by means of markers, and propagates the activation of white blood cells in the circulation.19
Development of varicose veins: the essential role of valvular alterations
It is not an alteration in the venous wall that induces damage to the valve but rather the opposite. Once valvular damage is done, it produces venous, and then cutaneous, damage. Nevertheless, varicose vein deformities will themselves promote development of the process, enhancing deterioration of the entire vessel (a vicious circle). Furthermore, progressive alteration of venous wall elasticity and contractility lead to extensive distension of the venous wall, because of the effect of VHT, which then secondarily alters functioning of the valves.
Damage to other valvular systems
The impact of valvular incompetence is variable, as explained by Vin (President of the French Society of Phlebology, personal communication-unpublished results), and its extent is quantified by duplex scanning.2 If dysplastic alterations develop opposite the ring of a valve located high in the saphenous veins, uncontrolled VHT will exert its effect from the upper to the lower part of the vein, producing segmental remodeling and progressive incompetence of the underlying valves. The valves thus damaged can be terminal valves, or in a collateral thigh vein. Distal, peripheral dilation can also develop, either in the reticular venous tissue dilating the subdermal veins, which then undergo remodeling, or by damage to the perforator veins (40% of cases of primary varicose veins). By a process of aspiration, the overlying saphenous veins become incompetent: the “bobsleigh” theory considers this to occur from the lower to the upper part of the vein.20 In this context, the ASVAL method (selective ablation of varicose veins under local anesthesia), which is undergoing evaluation, is designed to restore competence to the saphenous veins by phlebectomy of the distal collateral branches.2
Cutaneous tissue alterations
Elevated intensity and prolonged duration of VHT in lower limb veins carries the risk of skin disorders, since naive skin is not able to protect itself. An example of this is incompetent perforator venous valves in the juxtamalleolar area, which result in venous ulceration. Similarly, the classical relationship between a leg ulcer and elevated ambulatory venous pressure should be kept in mind. The disorders observed include increased capillary permeability with leakage and edema, capillary changes (Fagrell and Bollinger’s microangiopathy), and subsequently skin alterations with alternating inflammatory and atrophic areas. A leg ulcer is the result of these changes, but its course is slow, dissociated, and depends on additional factors. In a first phase, VHT induces mainly edema, with pain, an early sign of venous disease. Hemorheological disorders add to this process (enhanced red blood cell aggregation), due to fluid leakage (contraction of plasma volume), and elevated serum fibrinogen related to inflammation. The mechanisms have been defined by study of skin biopsies and center on the leukocyte/endothelium interaction. The endothelium of microvenules and subdermal plexuses expresses adhesion molecules (ICAM-1, vascular cell adhesion molecule-1 [VCAM-1]).21,22 Circulating leukocytes are activated and express integrin CD11b. The theory of white blood cell retention and activation proposed by Coleridge-Smith’s school (white cell trapping) is now recognized.23 It sheds light on the pathogenesis of an ulcer, which arises because white blood cells deliver to the epidermis enzymes active on the extracellular matrix, and vascular endothelial growth factor, which is active on capillary permeability.25
Damage to large veins: formation of a varicose vein by remodeling
A varicose vein develops as the result of degenerative transformation of the layers of the venous wall, which undergo remodeling (which means a “change in shape”). This process is associated with the leukocytes, which, under the influence of VHT, successfully pass into the venous wall, thereby producing hypoxia and alterations in shear stress.
Hypoxia (Figure 2) affects the sinus in the venous valves, but is also luminal in the presence of venous stasis,26 and medial by compression of the vasa vasorum.27 Hypoxia first affects the sinus, and as seen before is a constant finding in the valvular nidus. The other sites are progressively activated. Hypoxia activates the endothelium, which then expresses adhesion molecules.28
Anomalies of shear stress (Figure 4)
Normally, the endothelium lining the venous wall is sheared by laminar flow. Regular, and relatively high pressures keep the adhesive functions of the endothelial tissue dormant and promote homeostasis, in particular by the secretion of nitric oxide (NO).29 But the circulatory mechanisms form sites where these functions are lost. First and foremost, in the sinus where zones not subject to shear promote contact between leukocytes and endothelium. Then VHT, by virtue of a pressure difference and creation of turbulence, alters laminar flow along the walls of the veins, and forms subvalvular zones of recirculation, which are not subject to shear and also promote transport of cells.
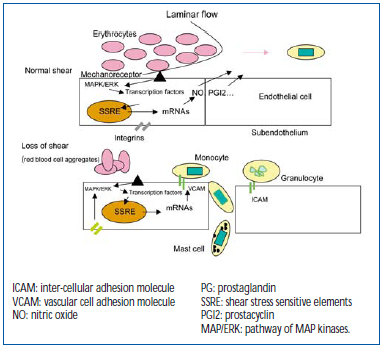
Figure 4. Laminar flow “shearing” of mechanoreceptors of the endothelium in the direction of protection of the blood vessel (10 dynes/cm2) re-inhibits molecular expression of adhesions, selectins, ICAM-1, and VCAM-1. Under abnormal conditions of blood flow producing a fall in shear stress (< 5 dynes/cm2), the mechanoreceptors induce the opposite effect. Many genes are sensitive to mechanoreceptors and to stretching of integrins.
Figure 4 depicts the mechanisms of mechanotransduction: from receptors sensitive to pressure variations exerted by circulating blood acting on MAP kinase signaling cascades, and then on transcription factors able to activate genes sensitive to stress, delivering a large number of products, including adhesion molecules. The front of the shear flow can be altered by red blood cell aggregates, leading to changes in shear stress, thus defining the rheological action.30 Stretching of integrins, which connect the cell to its subendothelium, has the same action as mechanoreceptors, explaining how stretching and distension of the venous wall impact on venous wall function (stretching). The latter explains the “mechanical” action of pronounced distension acquired after several years of remodeling.
Remodeling, the ultimate result
Figure 5 illustrates the processes that take place in the venous wall. Macroscopically, hypertrophic areas, where ECM components accumulate, alternate with atrophic areas containing few cells and fibers. Macroscopic lesions are characterized by an increase in rigid, type I collagen, and also a decrease in more distensible type III collagen, associated with rupture and rarefied elastin bundles.31 Intense cellular activity is observed among fibroblasts and smooth muscle cells. The latter, dedifferentiated, acquire secretory properties and migrate towards the thick intimal layer.32 Thus, the leukocytes are at work, with the neutrophils attached to the outer and inner layers of the endothelium, thus contributing to its high activation. Monocytes, macrophages, and T and B lymphocytes are observed in the tunica intima and media, and especially mast cells, whose abundance greatly increases.8 Apart from participation in remodeling, mast cells induce extensive activation because they contain high amounts of histamine and bradykinin, the basis for expression of endothelial selectins. Mast cells also play a part in pain, since they can activate the nociceptors of amyelinic C fibers. These changes in the ECM are related to enzyme systems associated with growth factors, which are transported and delivered by the smooth muscle cells and mast cells (Table I). Zn-dependent metalloproteinases act at an early stage. MMP 9 arises from granules in neutrophils activated at the paraendothelial level, and observed in varicose venous blood.33 MMPs 2 and 3 intervene later, are active in the media, to which they are transported by mast cells, and are secreted in situ by fibroblasts and smooth muscle cells. Other types only occur at CEAP stages 5 and 6.34 The MMPs are in contact with their TIMP inhibitor, whose balance is greatly upset during remodeling.35 The main growth factor present is TGF_1. It is transported by the mast cells and strongly activates the fibroblasts to produce fibers.36 PDGF and basal FGF are produced by the endothelial cells during hypoxia.26 In summary, the interaction of the cellular mechanisms and factors brought into play in the ECM is complex, variable, and progresses by fits and starts, affecting one venous segment more than another, but reaching macroscopic proportions, which may sometimes be considerable. It should be noted that the same mechanisms intervene as those that interact in the skin. An analogy can be made with atherogenesis in arteries. In summary, the link between a hemodynamic disorder due to dysfunction of venous valves, circulating blood cells, the venous wall, and biochemical processes is established with regard to the etiology of varicose veins, and supports the venous “elasticity” effect of older concepts, which are of course still relevant.37
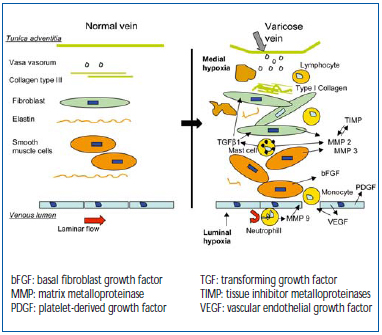
Figure 5. Interaction between blood cells associated with fibroblasts and smooth muscle cells during remodeling.
Table I. Cellular factors which participate in remodeling of the blood vessel wall (ECM – extracellular matrix; SMC – smooth muscle cells; MMP – matrix metalloproteinase; TIMP – tissue inhibitor of metalloproteinase).
These findings point to how alterations occur in the infiltrated valve, with remodeling of the vein. In contrast to the views of many authors, the primary event no longer lies in the wall of the vein. This does not prevent secondary major mechanical abnormalities in the vessel from worsening and even inducing alterations.
Variability of varices due to many precipitating factors
Varicose vein disease is expressed very differently from one person to another, in terms of familial background, gender, society, and lifestyle. This is not surprising when we consider the large number of factors that predispose to and promote varicose veins.
• Ethnic group: the differences observed tend to be related to types of physical activity, which in this context correspond to the role attributed to prolonged VHT.
• Age: acts through the duration factor.
• Female gender: the influence of hormones on the venous wall is plausible, although difficult to demonstrate, but women with varicose veins have a later menopause. The role of pregnancy is clear, and emphasizes the need for prevention by compression therapy.
• Height and weight: height is a demonstrated risk factor, while obesity is not an independent factor. Nevertheless, excess weight increases the risk of leg ulcer.
• Manual work: a relationship exists between VHT and uncomfortable physical activity in a prolonged standing position.
• Genetics: a family link has been confirmed in several studies, including a well-known investigation by Cornu-Thénard et al,38 who also reported links to blood group and HLA type.
Hereditary abnormalities in elastin are obviously a cause of varicose veins, as in Marfan disease. There are also abnormal lines of fibroblasts that secrete defective categories of collagen or elastin. Prothrombogenic conditions develop during the course of varicose vein disease, with a propensity to hypofibrinolysis and hypercoagulability.39,40
Secondary varices
Congenital valvular aplasia leads to varices, even though the predominant manifestation is edema. Arteriovenous shunts cause varices, differentiating them from development of vasa vasorum. Varices associated with angiodysplasia fall within the scope of Klippel- Trenauney and Parkes-Weber syndromes, with unilateral lower limb hypertrophy. Alternating channels are related to deep vein thrombosis, vascular agenesis, a gravid uterus, and tumors. Lastly, Cockett’s syndrome, and venous hyperoutput in athletes, act on venous pressure. In summary, all these causes, most of which are nevertheless rare, promote VHT, leukocyte adhesion, or remodeling, independently or in combination.
CONCLUSION
The demonstration of a leukocyte/endothelium cellular interaction is another step in elucidating the singular etiology of chronic venous disease. The micronized purified flavonoid fraction (MPFF at a dose of 500 mg) consisting of 90% diosmin and 10% hesperidin clearly acts on leukocyte adhesion in the arteriovenous fistula model in the rat.15 Similar action has been demonstrated in patients.41 Phlebotropic drugs produce effects that are partly related to leukocyte interactions.42 Such effects, which are well tolerated, are not associated with a risk of neutropenia, which has been an obstacle for other products. Phlebotropic drugs act on leukocyte “rolling,” ie, the recruitment of leukocytes, with no danger to patients.43
ACKNOWLEDGEMENTS
The author wishes to express his sincere thanks to Françoise Pitsch for her thoughtful review of the manuscript.
REFERENCES
2. Vin F. La maladie veineuse : une maladie inflammatoire ? Unpublished results (personal communication).
3. Nicolaides AN. Chronic venous disease and the leukocyte endothelium interaction: from symptoms to ulceration. Angiology. 2005;56:S11-S19.
4. Van Cleef. Images angioscopiques de thrombus et de lésions post thrombotiques de la saphène interne. Phlébologie. 1996;49:15-17.
5. Blanchemaison P. Intérêt de l’angioscopie veineuse dans l’exploration et le traitement de l’insuffisance veineuse des membres inférieurs. J Mal Vasc. 1992;17: 109-112.
6. Schultz-Ehrenburgh U, Weindorf N, Matthes U, Hirche H. Etude épidémiologique sur la pathogenèse des varices Etude de Bochum I-III. Artères et Veines. 1994;13:13-22.
7. Moffatts CJ, Franks PJ, Doherty DC, Martin R, Blewett R, Ross F. Prevalence of leg ulceration in a London population. QJM. 2004;97:431-437.
8. Cornwall JV, Dore CJ, Lewis JD. Leg ulcers: epidemiology and etiology. Br J Surg. 1986:73:693-696.
9. Boisseau MR. Valvules veineuses des membres inférieurs : problèmes hémodynamiques, biologiques et relations physiopathologiques. J Mal Vasc. 1997:22:122-127.
10. Hamer JD, Malone PC, SiIver IA. The PO2 in venous valve pockets: its possible bearing on thrombogenesis. Br J Surg. 1981;68:166-170.
11. Sewitt S. The structure and growth of valve pocket thrombi in femoral veins. J Clin Pathol. 1974;27:517-528.
12. Lurie F, Kistner RL, Eklof Bo, Kessler D. Mechanism of venous valve closure and role of the valve in circulation: a new concept. J Vasc Surg. 2003;38:955-961.
13. Van Bemmelen SP, Hoynck van Papendrecht AA, Hodde KC, Klopper PJ. A study of valve incompetence that developed in an experimental model of venous hypertension. Arch Surg. 1986;121:1048-1052.
14. Takase S, Pascarella L, Bergan JJ, Schmid- Shönbein GW. Hypertension induced venous valve remodeling. J Vasc Surg. 2004;39:1329-1334.
15. Takase S, Pascarella L, Lerond L, Bergan JJ, Schmid-Shönbein GW. Venous hypertension, inflammation and valve remodeling. Eur J Endovasc Surg. 2004;28:484-493.
16. Lorant M, Nadasy GL, Raffai G, Monos E. Remodeling of the rat saphenous vein network in response to long term gravitational load. Physiol Res. 2003;52: 525-531.
17. Ono T, Bergan JJ, Schmid-Shönbein GW, Takase S. Monocyte infiltration into venous valves. J Vasc Surg. 1998;27:158- 166.
18. Kakkos SK, Zolota VG, Peristeropoulos P, Apostolopoulou A, Geroulakos G, Tsolakis A. Increased mast cell infiltration in familial varicose veins: pathogenesis implications. Int Angiol. 2003;22:43-49.
19. Takase S, Schmid-Schönbein GW, Bergan JJ. Leukocyte activation in patients with venous insufficiency. J Vasc Surg. 1999;30:148-156.
20. Fegan WG. Varicose veins: the “bobsleigh” theory. Phlebology. 1993;8:142-144.
21. Peschen M, Lahaye T, Hennig B, Weyl A, Simon JC, Vanscheidt W. Expression of the adhesion molecules ICAM-1, VCAM-1, LFA-1 and VLA-4 in the skin is modulated in progressing stages of chronic venous insufficiency. Acta Derm Venereol. 1999;79:27-32.
22. Saharay M, Shields DA, Georgiannos SN, Porter JB, Scurr JH, Coleridge-Smith PD. Endothelial activation in patients with chronic venous disease. Eur J Vasc Endovasc Surg. 1998;15:342-349.
23. Coleridge-Smith PD, Thomas P, Scurr JH, Dormandy JA. Causes of venous ulceration: a new hypothesis. Br Med J. 1988;296:1726-1727.
24. Lal BK, Saito S, Pappas PJ, Padberg FT Jr, Cerveira JJ, Hobson RW 2nd, Duran WN. Altered proliferation responses of dermal fibroblasts to TGFbeta1 may contribute to chronic venous stasis ulcer. J Vasc Surg. 2003;37:1285-1293.
25. Lal BK, Varma S, Pappas PJ, Hobson RW2nd, Duran WN. VEGF increases permeability of the endothelial cell monolayer by activation of PKB/akt, endothelial nitric oxide synthase and MAP kinase pathways. Microvasc Res. 2001;62: 252-262.
26. Michiels C, Bouaziz N, Remacle J. Role of the endothelium and blood stasis in the development of varicose veins. Int Angiol. 2002;21:18-25.
27. Taccoen A, Lebard C, Borie H, Poullain JC, Zuccarelli F, Gerentes I, Stern S, Guichard M. Mesure de la pression en oxygène dans la paroi veineuse normale et anormale. J Mal Vasc. 1996;21:259-266.
28. Closse Ch, Seigneur M, Renard M, Pruvost A, Dumain P, Belloc F. Influence of hypoxia and hypoxia reoxygenation on endothelial P selectin expression. Thromb Res. 1997;85:159-164.
29. Alfke H, Kleb B, Klose KJ. Nitric oxide inhibits the basic fibroblast growth factor stimulated migration of bovine vascular smooth muscle cells in vitro. VASA. 2000;29:99-102.
30. Baskurt OK, Yalcin O, Ozdem S, Armstrong JK, Meiselman HJ. Modulation of endothelial nitric oxide synthase expression by red cell aggregation. Am J Physiol Heart Circ Physiol. 2004;286:H222- H229.
31. Sansilvestri-Morel P, Rupin A, Badier- Commander C, Kern O, Fabiani JN, Verbeuren TJ, Vanhoutte PM. Imbalance in the synthesis of collagen type I and collagen type III in smooth muscle cells derived from human varicose veins. J Vasc Surg. 2001;38:560-568.
32. Sansilvestri-Morel P, Rupin, Jullien ND, Lembrez N, Mestries-Dubois P, Fabiani JN, Verbeuren TJ. Decreased production of collagen type III in cultured smooth muscle cells from varicose veins patients is due to a degradation by MMPs, possible implication of MMP 3. J Vasc Res. 2005;42:388-398.
33. Pascarella L, Penn A, Schmid-Schönbein GW. Venous hypertension and the inflammatory cascade: major manifestations and trigger mechanisms. Angiology. 2005;56:S3-S10.
34. Saito S, Trovato MJ, You R, Lal BK, Faschum F, Padberg FT Jr, Hobson RW, Duran WN, Pappas PJ. Role of MMP 1, 2, and 9 and tissue inhibitor of MMP-1 in chronic venous insufficiency. J Vasc Surg. 2001;34:930-938.
35. Badier-Commander C, Verbeuren T, Lebard C, Michel JB, Jacob MP. Increased TIMP/MMP ratio in varicose veins: a possible explanation for extra cellular matrix accumulation. J Pathol. 2000;192:105-112.
36. Jacob T, Hingorani A, Ascher E. Overexpression of transforming growth factor beta 1 correlates with increased synthesis of nitric oxide synthase in varicose veins. J Vasc Surg. 2005;41:523- 530.
37. Clarke H, Smith SRG, Vasdekis SN, Hobbs JT, Nicolaides AN. Role of venous elasticity in the development of varicose veins. Br J Surg. 1989;76:577-580.
38. Cornu-Thénard A, Boivin P, Baud JM, De Vicenzy I, Carpentier PH. Importance of the familial factor in varicose disease. J Dermatol Surg Oncol. 1994;20:318-320.
39. Boisseau MR, Khodabandehlou, C Le Dévéhat. Fibrinolytic and biochemical factors in patients with venous insufficiency. Phlebology. 2004;19:7-13.
40. Pereira de Godoy JM, Andrale Torres CA, Braile DM. Prevalence of antithrombin deficiency in patients with chronic leg ulcer Blood Coagul Fibrinolysis. 2001;12:593-595.
41. Boisseau MR. Pharmacological targets of drugs employed in chronic venous and lymphatic insufficiency. Int Angiol. 2002; 21:33-39.
42. Shoab SS, Porter JB, Scurr JH, Coleridge- Smith PD. Effect of oral micronized purified flavonoid fraction treatment on leukocyte adhesion molecule expression in patients with chronic venous disease: a pilot study. J Vasc Surg. 2000;31:456-461.
43. Renard M, Heutte F, Boutherin-Falson O, Finet M, Boisseau MR. Induced changes of leukocyte slow rolling in an in flow pharmacological model of adhesion to endothelial cells. Biorheology. 2003;40: 173-178.